Battery Energy Storage Systems (BESS): How They Work, Key Components, Benefits, and Future Trends
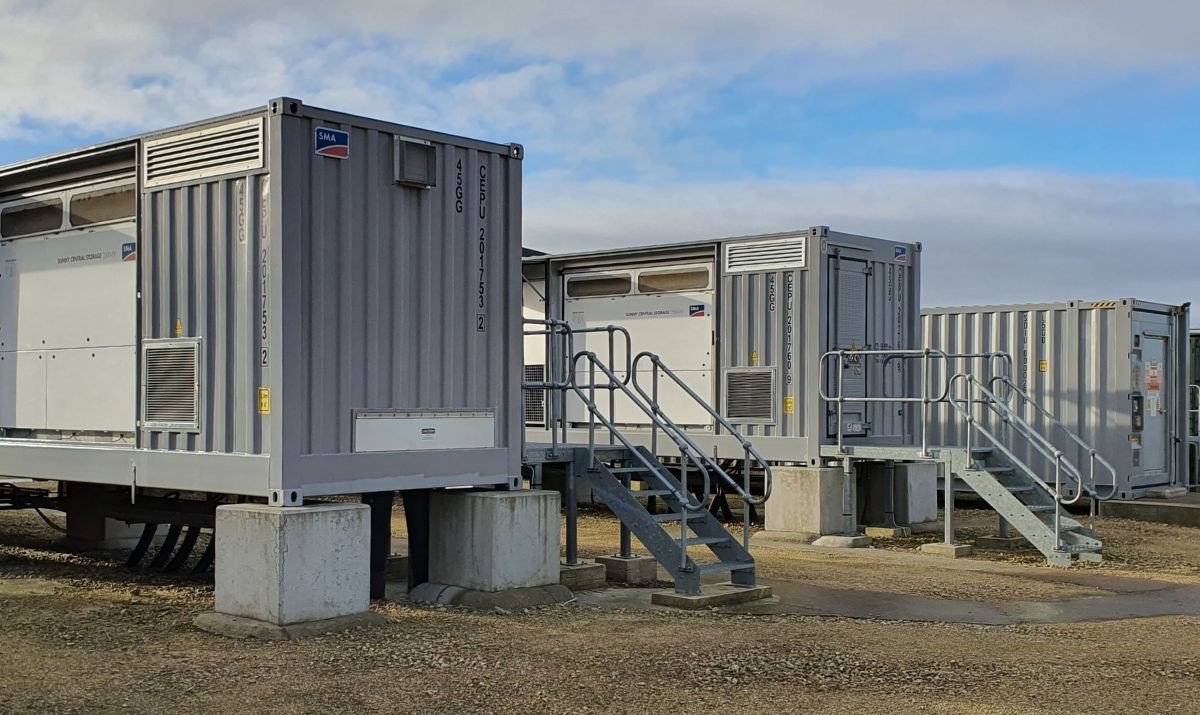
Over the past 20 years, BESS technology has advanced significantly in terms of storage capacity, battery chemistry, and system integration (Li et al., 2018; , Shen & Khaligh, 2015). But how exactly do these systems work, and why are they crucial to the future of energy?
This article gives a detailed account of battery storage systems, including how they operate, their key components, the financial and operational advantages they offer, and the trends that will define the future of energy storage. It provides useful information on how batteries operate and their place in the current energy landscape.
How Battery Energy Storage Systems Work
Battery storage systems operate using electrochemical principles—specifically, oxidation and reduction reactions in battery cells. During charging, electrical energy is converted into chemical energy and stored within the battery. When energy is needed, the system discharges, converting the chemical energy back into electricity for grid use or direct consumption (Li et al., 2022; , Park et al., 2022).
A key advantage of batteries is their rapid response time—they can charge and discharge almost instantly. This makes them ideal for grid services like frequency regulation, peak shaving, and load balancing. Such capabilities are especially valuable in energy systems with high levels of Variable Renewable Energy Sources (VRES), such as wind and solar power, which are inherently intermittent (Lei & Gong, 2017; , Kurtoğlu & Eroğlu, 2024).
They come in different configurations tailored to specific energy and power needs. Their modular and flexible designs allow them to be deployed across various settings, including residential, commercial, utility-scale projects, and microgrids.

Main Components of BESS
Understanding how BESS function requires a breakdown of their key components:
1. Battery Cells
Battery cells are the core of any storage system, where the actual energy conversion takes place. Lithium-ion batteries are the most common due to their high energy density and efficiency. However, other chemistries—like lead-acid, sodium-sulfur, and flow batteries—offer benefits in applications that require long lifespans or high thermal tolerance (Chen et al., 2023; , Zhang et al., 2016).
2. Battery Management System (BMS)
The BMS monitors and controls battery performance, ensuring safe operation by tracking variables like voltage, temperature, current, and State of Charge (SoC). Advanced BMS platforms use predictive algorithms to extend battery life and optimize performance.
3. Power Conditioning System (PCS)
The PCS manages the electrical interface between the battery and the power grid. It converts the direct current (DC) produced by batteries into alternating current (AC), enables bidirectional energy flow, and ensures compliance with grid specifications.
4. Inverters
Inverters convert DC energy stored in batteries to grid-compatible AC energy. They regulate output, maintain safety limits, and ensure the system complies with grid standards.
5. Cooling Systems
Thermal management is essential to prevent overheating, which can degrade performance and safety. Cooling systems help maintain optimal temperatures, improving reliability and extending the lifespan of battery components.
6. Communication Interfaces
Modern batteries feature communication systems that interact with grid operators, enabling real-time monitoring and remote control. These interfaces support multiple protocols to ensure compatibility and system-wide coordination (Chen & Wang, 2024).
Financial Benefits of Battery Energy Storage Systems
As battery costs decline and energy resilience becomes a higher priority, battery systems are increasingly attractive for their economic advantages (Li et al., 2021):
1. Reduced Costs via Demand Response
Batteries enable strategic energy load shifting, allowing participation in demand response programs. Businesses can cut energy bills by avoiding peak prices.
2. New Revenue Streams
This technology can generate revenue by participating in ancillary services and energy arbitrage—buying energy when prices are low and selling it during peak demand.
3. Infrastructure Cost Savings
By alleviating grid congestion and delaying the need for new infrastructure, battery storage systems help utilities manage growing energy demands more cost-effectively.
4. Reliable Backup Power
Batteries provide dependable backup power during outages. For many organizations, uninterrupted operations justify the investment in energy storage.
5. Enhanced Renewable Energy Integration
Batteries improve the reliability of solar and wind projects by managing their variability, increasing project bankability and accelerating renewable adoption.
Operational Benefits of BESS
Beyond financial value, battery storage systems offer several key operational advantages:
1. Improved Grid Stability
By balancing supply and demand, batteries help prevent voltage and frequency disruptions, strengthening overall grid reliability.
2. Greater Operational Flexibility
Their ability to respond quickly to shifting energy needs gives system operators more control and agility in energy management.
3. Lower Carbon Emissions
By reducing reliance on fossil fuel-based peaker plants, they contribute to cleaner energy systems and support climate goals.
4. Electrification Support
Batteries play a critical role in the electrification of transportation and heating, helping industries transition away from fossil fuels.
5. Enablement of Microgrids
The modular nature of battery systems supports the creation of self-sustaining microgrids, particularly valuable in remote or outage-prone areas.
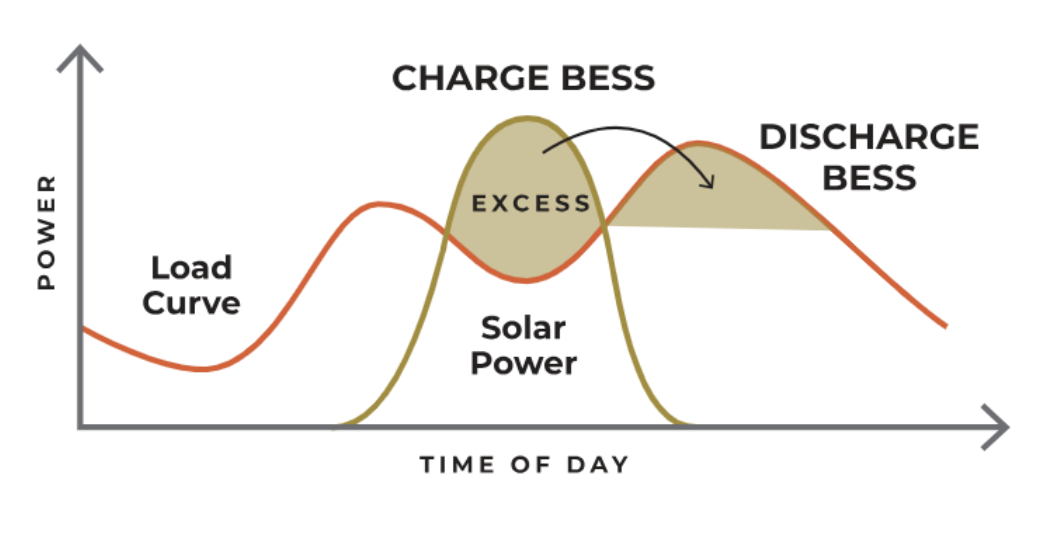
Innovations in Hybrid Energy Storage Systems
A growing innovation trend is Hybrid Energy Storage Systems (HESS), which combine batteries with technologies like supercapacitors. While batteries store large amounts of energy for longer durations, supercapacitors excel in fast charge/discharge cycles. The combination enhances performance, efficiency, and reliability across applications—especially in solar and wind power systems (Ahmed G. Abo-Khalil et al., 2023).
Future Trends in Battery Energy Storage
The future of BESS is being shaped by several key trends:
1. Technological Advancements
Next-generation technologies like solid-state batteries promise better safety, higher energy density, and longer life spans, potentially reducing overall system costs.
2. AI-Driven Optimization
Artificial intelligence and machine learning are being integrated into energy management systems to enable real-time monitoring, predictive maintenance, and optimal performance.
3. Sustainability and Recycling
With increased scrutiny on environmental impacts, the industry is focusing on battery recycling, second-life applications, and circular economy strategies.
4. Supportive Regulations
Governments worldwide are introducing incentives, clearer regulatory frameworks, and market mechanisms that encourage battery adoption.
5. Growth in Emerging Markets
As battery storage systems become more affordable, developing economies with energy access challenges present significant opportunities for battery systems deployment.
6. Expansion of HESS
The adoption of hybrid systems will likely increase, providing flexible solutions by combining the strengths of different energy storage technologies.
It's worth highlighting that Delfos Energy is currently expanding its capabilities in energy storage monitoring with a new solution designed specifically for Battery Energy Storage Systems (BESS). Focused on supporting asset managers in the wind and solar sectors, the platform enables advanced analytics for performance auditing and operational optimization of storage assets.
Conclusion
Battery Energy Storage Systems present a compelling and dynamic approach to managing energy resources effectively. Their multifaceted functions allow for remarkable flexibility, resilience, and sustainability in diverse applications across the energy sector. With continued advancements in technology, the financial landscape shifting towards renewable energy integration, and heightened recognition of the importance of energy storage, battery storage systems are anchored as a cornerstone of future energy strategies.
As experts in the energy industry navigate the complexities of transition challenges, understanding the comprehensive nature of battery storage systems will be critical in harnessing their full potential for the forthcoming energy landscape. Batteries not only embodies the transition towards cleaner energy use but also promotes economic and operational viability crucial for a sustainable energy future.
In sum, the expansion of battery storage systems not only marks a technological milestone in modern energy management but serves as a prelude of a fundamentally different energy paradigm that values resilience, sustainability, and economic viability. Such transformation is not simply an evolution; it is a structural necessity for adapting to the changing contours of global energy dynamics.
Interested in how BESS can transform your energy strategy? Contact us for a demo or subscribe to our newsletter for more insights.
Bibliography
Li, X., Chalvatzis, K., & Stephanides, P. (2018). Innovative energy islands: life-cycle cost-benefit analysis for battery energy storage. Sustainability, 10(10), 3371. https://doi.org/10.3390/su10103371
Shen, J. and Khaligh, A. (2015). A supervisory energy management control strategy in a battery/ultracapacitor hybrid energy storage system. IEEE Transactions on Transportation Electrification, 1(3), 223-231. https://doi.org/10.1109/tte.2015.2464690
Li, X., Ma, R., Wei, G., & Yan, S. (2022). Optimal dispatch for battery energy storage station in distribution network considering voltage distribution improvement and peak load shifting. Journal of Modern Power Systems and Clean Energy, 10(1), 131-139. https://doi.org/10.35833/mpce.2020.000183
Park, J., Choi, J., Jo, H., Kodaira, D., Han, S., & Acquah, M. A. (2022). Life evaluation of battery energy system for frequency regulation using wear density function. Energies, 15(21), 8071. https://doi.org/10.3390/en15218071
Lei, J. and Gong, Q. (2017). Operating strategy and optimal allocation of large‐scale vrb energy storage system in active distribution networks for solar/wind power applications. IET Generation, Transmission &Amp; Distribution, 11(9), 2403-2411. https://doi.org/10.1049/iet-gtd.2016.2076
Kurtoğlu, M. and Eroğlu, F. (2024). Design and simulation of bidirectional dc-dc converter topology for battery applications. E3S Web of Conferences, 551, 03002. https://doi.org/10.1051/e3sconf/202455103002
Chen, X., Hua, J., Yin, C., Chen, Z., Chen, Y., & Li, Z. (2023). A precise life estimation method for retired energy storage batteries based on energy storage batteries attenuation characteristics and xgboost algorithm. IEEE Access, 11, 135968-135978. https://doi.org/10.1109/access.2023.3336813
Zhang, Y., Dong, Z. Y., Luo, F., Zheng, Y., Meng, K., & Wong, K. P. (2016). Optimal allocation of battery energy storage systems in distribution networks with high wind power penetration. IET Renewable Power Generation, 10(8), 1105-1113. https://doi.org/10.1049/iet-rpg.2015.0542
Chen, Z. and Wang, B. (2024). Design of ship power system with exchangeable battery energy storage containers. Ninth International Symposium on Sensors, Mechatronics, and Automation System (ISSMAS 2023). https://doi.org/10.1117/12.3014886
Li, X., Wang, Q., Chen, Y., Li, Y., He, Z., Wang, T., … & Wu, X. (2021). Safety analysis of energy storage station based on dfmea. E3S Web of Conferences, 236, 01006. https://doi.org/10.1051/e3sconf/202123601006
Ahmed G. Abo-Khalil, Ahmed Sobhy, Mohammad Ali Abdelkareem, A.G. Olabi, Advancements and challenges in hybrid energy storage systems: Components, control strategies, and future directions, International Journal of Thermofluids, Volume 20, 2023, 100477, ISSN 2666-2027, https://doi.org/10.1016/j.ijft.2023.100477.
Book a meeting
Let's connect and forge new partnerships
Custom Renewable Energy Solutions
Contact us today to discuss your renewable energy needs and find the perfect solution for your business.