Reuse, recycling and disposal of wind turbine parts: An investigation into industry practices

The proper disposal, recycling and reuse of such parts are essential to preserve the environmental advantages of wind power since decommissioned turbines generate substantial waste. This article investigates current industry practices regarding the wind turbine generator (WTG) waste management, with a focus on blades, which are the most challenging components to manage at the end of their life cycle.
Wind Turbine Parts
Wind turbines are intricate machines designed to convert the kinetic energy of wind into electrical energy. They comprise several essential components that work together seamlessly to optimize energy production. The principal parts of a modern wind turbine include the rotor, nacelle, tower, and various electronic and mechanical systems, each playing a critical role in the turbine's operation.
Rotor
The rotor consists of the blades and hub, which are responsible for capturing wind energy. The blades can be adjusted (pitched) and are designed with control surfaces to enhance performance. Materials used for the blades include fiberglass, wood, and aluminum, with advanced composites providing a balance of strength and lightness. A typical fiberglass blade can be around 15 meters long and weigh up to 2,500 pounds (approximately 1.134 kg), depending on the size, model and material of the component.

Nacelle
The nacelle is a protective housing situated atop the tower, containing essential components such as the main drive shaft, gearbox, generator, and power electronics. Typically made from fiberglass, it also includes systems for blade pitch control and yaw control, which adjust the turbine's orientation relative to the wind. A typical nacelle weighs around 22,000 pounds (approx. 9.979 kg) and contains various mechanical and electronic parts crucial for the turbine's efficiency.

Tower
The tower supports the nacelle and rotor at a height that maximizes wind exposure. Towers are often constructed from galvanized steel or concrete, providing stability and durability against harsh environmental conditions. Their design can vary, including tubular or lattice structures, depending on the application and required height.
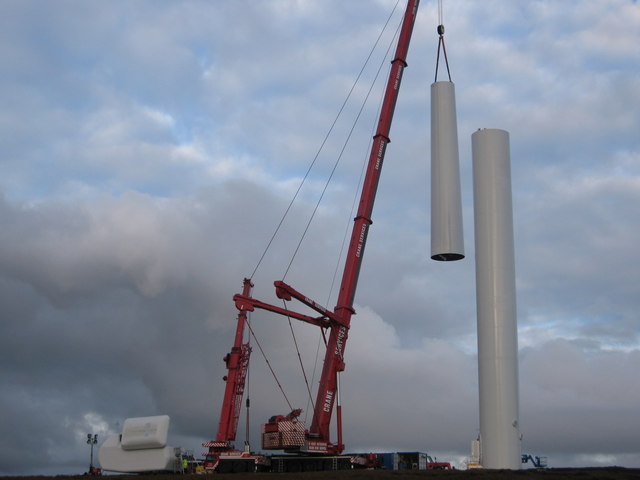
Drive Train
The drive train comprises several key components, including the low-speed shaft (LSS), bearings, couplings, gearbox, high-speed shaft (HSS), and brakes. This system converts the mechanical energy captured by the rotor into electrical energy through the generator. Advanced drive train configurations are essential for optimizing the conversion process and ensuring reliability.
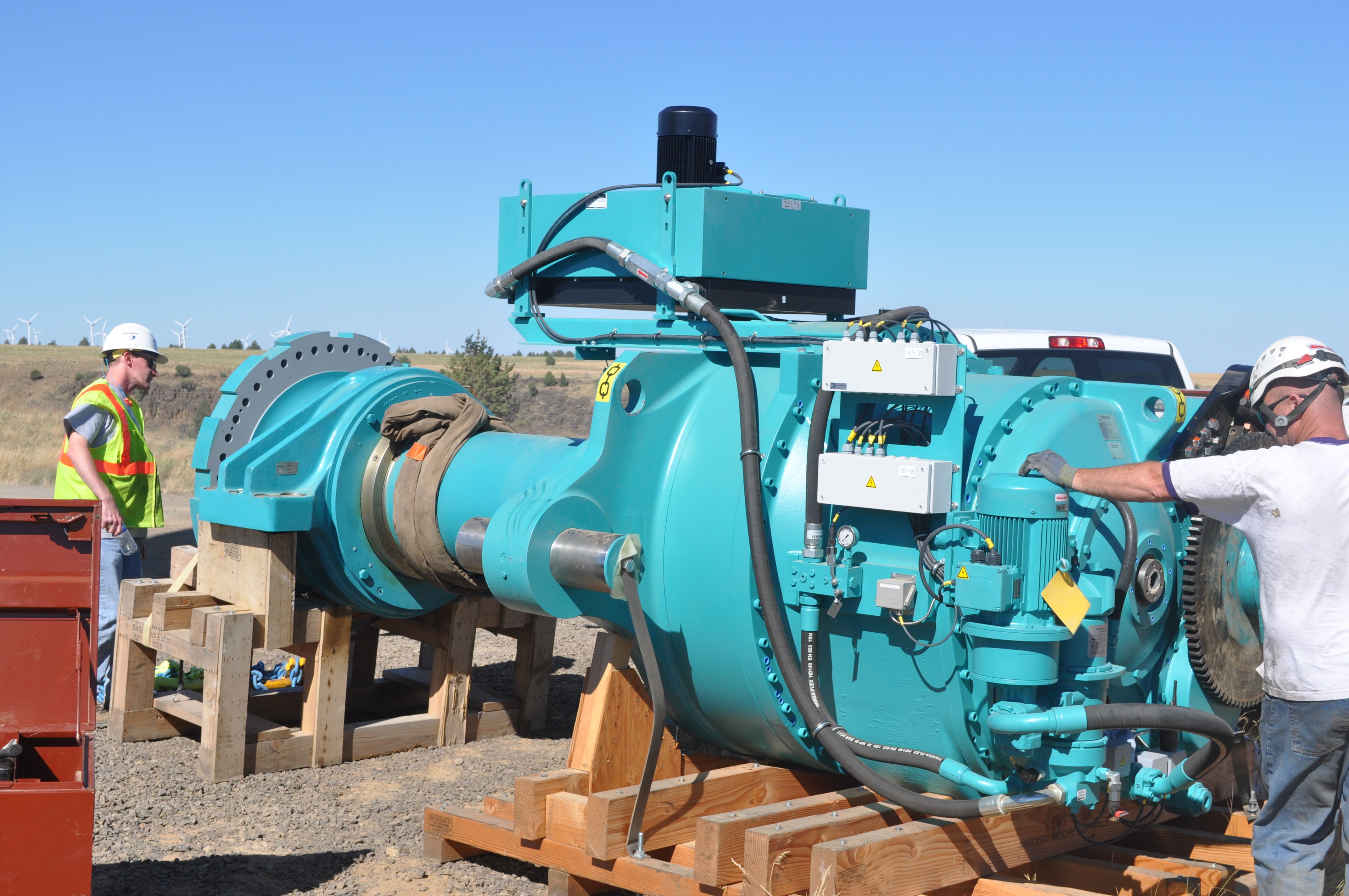
Power Electronics
The power electronics interface acts as the “brain” of the wind turbine, managing the flow of electricity generated and optimizing performance. It includes power converters that enable control over active and reactive power, ensuring efficient integration with the electrical grid. The interface decouples the rotational speed of the wind turbine from the electrical frequency, allowing for variable-speed operation.
Additional Components
Other vital components include transformers, which convert the generated low-voltage electricity to a medium voltage level to minimize transmission losses, and utility boxes that facilitate the overall energy conversion process. The complexity and variety of materials used in these components, including steel, copper, and rare Earth elements, highlight the advancements in technology aimed at improving wind energy efficiency and sustainability.
The manufacturing process of wind turbine blades contributes significantly to waste generation. According to researchers, 10-15% of the used materials are wasted during blade fabrication and then disposed of in landfills (Almeida et al., 2022). Most of the waste produced is made of composite materials, which are hard to recycle because of their structure. This problem is highlighted by the fact that every year tens of thousands of tons of wind turbine waste are generated from decommissioned blades (Kalman, 2021). The industry must adopt comprehensive recycling solutions to mitigate the environmental impact of this waste, as incineration contributes to CO2 emissions and exacerbates climate change (Kalman, 2021).
In response to the growing waste problem, various initiatives and projects have been launched to enhance the recyclability of wind turbine blades. For instance, the ZEBRA project aims to develop 100% recyclable composite blades, while the DecomBlades project explores sustainable recycling techniques (Yang et al., 2023). These initiatives highlight a shift towards a circular economy in the wind energy sector, where the focus is on reusing materials and minimizing waste. However, the current recycling technologies are still in their infancy, and significant advancements are needed to transition from laboratory-scale solutions to commercial applications (Chen et al., 2019).
Due to the composition of the wind turbine blades, recycling them becomes a problem. A majority of blades are made of glass fibre reinforced polymers (GFRP) whose components are not easily recyclable. At present, the recycling techniques are mechanical, thermal and chemical recycling, which have their own benefits and drawbacks. For instance, mechanical recycling entails shredding the blades into smaller pieces which can be employed as filler in other products. However, this approach makes the material quality deteriorate. Thermal recycling, on the other hand, can generate energy from the waste materials but may emit harmful pollutants if not disposed properly (Sorte et al., 2023).
Studies have investigated modern recycling approaches and discovered plasma technology which transforms wind turbine blades into microfibers according to the research by Kavaliauskas et al. (2023). The approach combines material recycling with new product creation which serves multiple applications across construction and manufacturing industries. Pyrolysis now stands out as an emerging effective approach that turns glass fibre composite materials into high-standard fibers which find their way into fresh production materials (Ma et al., 2024). The wind energy sector now shows increased awareness about sustainable practices because of these developments.
The environmental impact of wind turbine waste extends beyond the blades themselves. The concrete foundations and metal components of turbines also pose disposal challenges. As the number of decommissioned turbines increases, the volume of waste generated is expected to grow exponentially, with projections suggesting that over 200,000 tons of blade waste could be produced by 2034 (Hao et al., 2020). This underscores the importance of developing comprehensive end-of-life (EoL) management strategies that encompass all components of wind turbines, not just the blades.
The environmental impacts of wind turbine waste management practices have been evaluated through life cycle assessments (LCA). The environmental costs of waste disposal are significant although wind turbines produce significantly more energy than what is spent on their manufacturing according to Paulsen and Enevoldsen (2021). According to recent assessments the total environmental losses from a single wind turbine range between EUR 500,000 and EUR 1.1 million. Thus, there is a clear need for proper waste management plans. As for now, the recyclability of the current wind turbine designs is believed to be 86%, which means that the material recovery can still be enhanced (Paulsen & Enevoldsen, 2021).
Challenges in Recycling and Reuse
The recycling and reuse of wind turbine components, particularly the blades, present significant challenges due to their complex materials and large sizes. As the industry grapples with the end-of-life phase of wind energy systems, these challenges become increasingly prominent.
Complexity of Materials
Wind turbine blades are primarily made from composite materials, such as fiberglass and epoxy resins, which complicate traditional recycling processes. These materials are designed for durability and longevity, making their disassembly and recycling difficult. Mechanical recycling methods, such as shredding, can produce lower quality materials, restricting their future applications in industry and construction. Moreover, advanced recycling techniques like High-Voltage Fragmentation (HVF) can yield cleaner fibers but require significant energy consumption, raising cost concerns. In the study by Mishnaevsky Jr. (2021), the author, in addition to investigating the most widely used techniques for reusing wind blades, analyzes alternative materials for their construction, such as natural fibers and bamboo, but stops short of using them in large-scale wind turbines.
Regulatory and Economic Factors
The implementation of effective recycling practices is also hindered by regulatory and economic barriers. Different countries have varying regulations regarding the disposal and recycling of wind turbine components, which can impact the development of cohesive recycling strategies. In regions like the European Union, regulations are adapting to address waste concerns from wind energy, yet challenges remain in enforcing these policies across member states. Additionally, the relative cost and value of recycling technologies vary widely, creating discrepancies in the feasibility of recycling practices among different companies and regions.
Infrastructure and Technological Gaps
The infrastructure required for efficient recycling is often lacking, especially in regions where wind energy is rapidly expanding. The establishment of recycling facilities is crucial for managing waste effectively; however, strategic siting and investment in recovery and recycling infrastructure remain limited. This situation requires collaboration between small and medium-sized enterprises and large corporations to innovate and improve waste management processes. Public policies aimed at promoting a circular economy can help, but they must be robustly supported to ensure they lead to tangible improvements in recycling practices.
Economic Viability
The economic viability of recycling wind turbine components is also a significant hurdle. Decommissioning costs can range between $114,000 and $195,000 per turbine, and while salvage estimates can reduce these costs, the overall economic framework for recycling remains underdeveloped. As a result, many developers may prioritize cost over environmentally sustainable practices, further complicating efforts to enhance recycling and reuse in the industry.
The integration of a circular economy approach into the wind energy sector could yield significant environmental savings. Implementing strategies that prioritize reuse and recycling can potentially achieve 20-30% reductions in environmental impacts associated with WTG waste. However, the transition to a circular economy is fraught with challenges, including the need for robust data on waste generation, the development of industrial-scale recycling technologies, and supportive policies that incentivize sustainable practices (Woo & Whale, 2022).
The challenges of turbine waste management require both technological advancements and collaboration between industry and academia. Innovation through stakeholder sector collaboration is enhanced when research initiatives are established to develop new solutions while promoting best practices. Through the university industry partnerships, new recycling processes have been discovered and pilot projects created to test their feasibility according to Astolfi et al.'s (2018) study.
There are different processes for reusing wind blades, most commonly mechanical methods, many of which have already been banned in many countries. Techniques that involve more refined chemical processing for the reuse of blades, such as pyrolysis and solvolysis, not only come up against the impossibility of large-scale application, but also the fact that blades can have different compositions, making it difficult to standardize techniques that need more refinement (Paulsen, 2020).
The regulatory landscape surrounding WTG waste management is also evolving. As the volume of decommissioned equipments increases, governments are beginning to implement policies aimed at promoting recycling and reducing landfill use. These policies may include mandates for recycling rates, financial incentives for companies that invest in sustainable practices, and support for research and development in waste management technologies (Mishnaevsky, 2021). Such regulatory frameworks will be essential for driving the transition towards a more sustainable wind energy sector.
Despite the progress made in recycling technologies and practices, significant barriers remain. The high costs associated with recycling processes, coupled with the low market value of recycled materials, can deter investment in sustainable waste management solutions (Sorte et al., 2023). Additionally, the lack of standardized recycling protocols and the variability in blade designs complicate the development of universal recycling methods (Sorte et al., 2023). Addressing these challenges will require concerted efforts from industry stakeholders, researchers, and policymakers to create a cohesive strategy for managing wind turbine waste.
Future Trends
As the renewable energy sector continues to evolve, the recycling and disposal of wind turbine components are becoming increasingly critical. The anticipated retirement of first- and second-generation wind turbines, alongside a growing number of new installations, underscores the necessity for effective recycling solutions to manage the resulting waste and resource utilization. According to Liu and Barlow (2017), it is estimated that by 2050, for every ton of material used to manufacture wind turbines, 0.7 tons of waste will be produced, meaning that by 2050 we will have 2.5 million tons of waste from the wind segment.
Advancements in Recycling Technologies
Significant advancements in recycling technologies are on the horizon. Industry experts are developing innovative methods to reclaim valuable materials from decommissioned wind turbine parts. For instance, companies are exploring techniques to break down composite materials into reusable raw materials, effectively closing the loop on what was once considered waste. This shift towards a circular economy is not limited to blades alone; refurbishing gearboxes and other metallic components for re-entry into the production cycle is gaining traction as a means to substantially reduce emissions.
Policy and Economic Incentives
Policy changes are expected to play a vital role in enhancing recycling efforts. Legislative mandates for the recycling of wind turbine components, coupled with financial incentives for companies that invest in recycling infrastructure, could accelerate technological advancements within the industry. Such initiatives will not only promote sustainability but also encourage economic growth through the creation of new jobs in the recycling and manufacturing sectors.
Collaborative Research Initiatives
Collaborative research efforts are critical for overcoming existing barriers to recycling. Establishing partnerships among research organizations, component manufacturers, and wind plant developers can facilitate the development of certification standards and methodologies for recycled materials, ensuring their acceptance in secondary markets. These collaborative efforts aim to qualify new sustainable materials, fostering innovation and advancing technologies to meet the growing demands of the wind energy sector.
Environmental Considerations
The focus on sustainability and innovation in wind turbine recycling is poised to contribute significantly to environmental stewardship. By implementing effective recycling processes, the industry can mitigate the risks of waste buildup and pollution associated with conventional disposal methods, thereby supporting a cleaner environment. The integration of bio-based components and thermoplastic polymers into new wind turbine designs is also expected to enhance the overall sustainability of materials used in the sector, ultimately influencing their recovery and recyclability in the future.
Conclusion
In conclusion, the reuse, recycling, and disposal of wind turbine parts are critical components of sustainable wind energy practices. As the industry faces an increasing volume of decommissioned turbines, it is imperative to develop effective waste management strategies that prioritize recycling and minimize environmental impacts. While significant advancements have been made in recycling technologies and practices, ongoing research, collaboration, and supportive policies will be essential for overcoming the challenges associated with wind turbine waste management. By embracing a circular economy approach, the wind energy sector can ensure that its environmental benefits are not overshadowed by the waste generated from decommissioned turbines.
References
- Almeida, E., Júnior, M., & Medeiros, D. (2022). Wind turbine blade manufacturing: a material flow analysis.. https://doi.org/10.5151/siintec2022-245225
- Astolfi, D., Castellani, F., & Terzi, L. (2018). Wind turbine power curve upgrades.. https://doi.org/10.20944/preprints201804.0269.v1
- Chen, J., Wang, J., & Ni, A. (2019). Recycling and reuse of composite materials for wind turbine blades: an overview. Journal of Reinforced Plastics and Composites, 38(12), 567-577. https://doi.org/10.1177/0731684419833470
- Hao, S., Kuah, A., Rudd, C., Wong, K., Lai, N., Mao, J., … & Liu, X. (2020). A circular economy approach to green energy: wind turbine, waste, and material recovery. The Science of the Total Environment, 702, 135054. https://doi.org/10.1016/j.scitotenv.2019.135054
- Kalman, J. (2021). Development of a polymer extrusion system to manufacture recycled bioplastic composites for additive manufacturing.. https://doi.org/10.32920/ryerson.14639052
- Kavaliauskas, Ž., Kėželis, R., Grigaitienė, V., Marcinauskas, L., Milieška, M., Valinčius, V., … & Baltušnikas, A. (2023). Recycling of wind turbine blades into microfiber using plasma technology. Materials, 16(8), 3089. https://doi.org/10.3390/ma16083089
- LIU, P.; MENG, F.; BARLOW, C. Y. Wind turbine blade end-of-life options: An eco-audit comparison. Journal of Cleaner Production, v. 212, p. 1268–1281, mar. 2019.
MATIVENGA, P. T., SHUAIB, N. A., HOWARTH, J., PESTALOZZI, F., WOIDASKY, J. High voltage fragmentation and mechanical recycling of glass fibre thermoset composite. CIRP Annals - Manufacturing Technology, v. 65, p. 45-48, 2016. https://doi.org/10.1016/j.jclepro.2018.12.043
- Ma, Y., Du, Z., Fang, X., Chen, B., Shi, L., & Li, H. (2024). Recycling glass fiber from polyurethane composite by pyrolysis strategy with high mechanical properties. Journal of Physics Conference Series, 2671(1), 012023. https://doi.org/10.1088/1742-6596/2671/1/012023
- Mishnaevsky, L. (2021). Sustainable end-of-life management of wind turbine blades: overview of current and coming solutions. Materials, 14(5), 1124. https://doi.org/10.3390/ma14051124
- Paulsen, E. and Enevoldsen, P. (2021). A multidisciplinary review of recycling methods for end-of-life wind turbine blades. Energies, 14(14), 4247. https://doi.org/10.3390/en14144247
- Sorte, S., Martins, N., Oliveira, M., Vela, G., & Relvas, C. (2023). Unlocking the potential of wind turbine blade recycling: assessing techniques and metrics for sustainability. Energies, 16(22), 7624. https://doi.org/10.3390/en16227624
- Woo, S. and Whale, J. (2022). A mini-review of end-of-life management of wind turbines: current practices and closing the circular economy gap. Waste Management & Research the Journal for a Sustainable Circular Economy, 40(12), 1730-1744. https://doi.org/10.1177/0734242x221105434
- Yang, J., Meng, F., Zhang, L., McKechnie, J., Chang, Y., Ma, B., … & Cullen, J. (2023). Solutions for recycling emerging wind turbine blade waste in China are not yet effective. Communications Earth & Environment, 4(1). https://doi.org/10.1038/s43247-023-01104-w
Book a meeting
Let's connect and forge new partnerships
Custom Renewable Energy Solutions
Contact us today to discuss your renewable energy needs and find the perfect solution for your business.